United States Patent |
4,858,612
|
Stocklin
|
August 22, 1989
|
Hearing device
Abstract
A method and apparatus for simulation of hearing in mammals by introduction
of a plurality of microwaves into the region of the auditory cortex is
shown and described. A microphone is used to transform sound signals into
electrical signals which are in turn analyzed and processed to provide
controls for generating a plurality of microwave signals at different
frequencies. The multifrequency microwaves are then applied to the brain
in the region of the auditory cortex. By this method sounds are perceived
by the mammal which are representative of the original sound received by
the microphone.
Inventors:
|
Stocklin; Philip L. (P.O. Box 2111, Satellite Beach, FL 32937)
|
Appl. No.:
|
562742 |
Filed:
|
December 19, 1983 |
Current U.S. Class: |
607/45; 607/56 |
Intern'l Class: |
A61N 001/36 |
Field of Search: |
128/419 R,419 S,422,653,771,732,741,746,791,804
340/407
|
References Cited [Referenced By]
U.S. Patent Documents
3490458 | Jan., 1970 | Allison | 128/421.
|
3751605 | Aug., 1973 | Michelson | 128/1.
|
3951134 | Apr., 1976 | Malech | 128/131.
|
4428377 | Jan., 1984 | Zollner et al. | 128/419.
|
Foreign Patent Documents |
893311 | Feb., 1972 | CA | 128/422.
|
2811120 | Sep., 1978 | DE | 128/419.
|
591196 | Jan., 1978 | SU | 128/419.
|
Other References
Gerkin, G., "Electroencephalography & Clinical Neurophysiology", vol. 135,
No. 6, Dec. 1973, pp. 652-653.
Frye et al., "Science", vol. 181, Jul. 27, 1973, pp. 356-358.
Bise, William, "Low Power Radio-Frequency and Microwave Effects on Human
Electroencephalogram and Behavior", Physiol. Chem. & Physics 10 (1978).
|
Primary Examiner: Kamm; William E.
Attorney, Agent or Firm: Wegner & Bretschneider
Claims
What is claimed is:
1. A sound perception device for providing induced perception of sound into
a mammalian brain comprising in combination:
means for generating microwave radiation which is representative of a sound
to be perceived, said means for generating including means for generating
a simultaneous plurality of microwave radiation frequencies and means for
adjusting the amplitude of said microwave radiation frequencies in
accordance with the sound to be perceived; and
antenna means located in the region of the auditory cortex of said
mammalian brain for transmitting said microwave energy into the auditory
cortex region of said brain.
2. A hearing device for perception of sounds comprising in combination:
means for generating a signal representative of sounds;
means for analyzing said signal representative of said sounds having an
output;
means for generating a plurality of microwave signals having different
frequencies having a input connected to said output of said means for
analyzing said signals, having an output;
means for applying said plurality of microwave signals to the head of a
subject, and
whereby the subject perceives sounds which are representative of said
sounds.
3. The apparatus in accordance with claim 2 wherein said means for
generating a signal is a microphone for detecting sound waves.
4. The apparatus in accordance with claim 2 wherein said means for applying
said plurality of microwave signals is an antenna.
5. The apparatus in accordance with claim 4 wherein said antenna is placed
in the region of the auditory cortex of the subject.
6. The apparatus in accordance with claim 2 wherein the subject is a human
being.
7. The apparatus in accordance with claim 2 wherein said means for
analyzing said signal comprises:
an acoustic filter bank for dividing said sounds into a plurality of
component frequencies; and
a mode control matrix means for providing control signals which are
weighted in accordance with said plurality of component frequencies,
having an output connected to said means for generating a plurality of
microwave signal inputs.
8. The apparatus in accordance with claim 7 wherein said acoustic filter
bank includes a plurality of audio frequency filters.
9. The apparatus in accordance with claim 8 wherein said audio frequency
filters provide a plurality of output frequencies having amplitudes which
are a function of said signal representative of sounds.
10. The apparatus in accordance with claim 9 wherein said amplitudes are
the weighted in accordance with transform function of the signal
representative of sounds.
11. The apparatus in accordance with claim 7 wherein said mode control
matrix device includes a voltage divider connected to each of said
plurality of said audio frequency filters.
12. The apparatus in accordance with claim 11 wherein each of said voltage
dividers has a plurality of outputs which are connected in circuit to said
means for generating a plurality of microwave signals.
13. The apparatus in accordance with claim 2 wherein said means for
generating a plurality of microwave signals comprises a plurality of
microwave generators each having a different frequency and means for
controlling the output amplitude of each of said generators.
14. The apparatus in accordance with claims 2 wherein said means for
generating a plurality of microwave signals comprises a broad band
microwave source and a plurality of filters.
15. The apparatus in accordance with claim 13 wherein said generators each
comprise a microwave signal source and a gain controlled microwave
amplifier.
16. The apparatus in accordance with claim 13 wherein said means for
analyzing output is connected to said means for controlling microwave
amplifier output amplitudes.
17. The apparatus in accordance with claim 13 wherein analyzing includes K
audio frequency filters.
18. The apparatus in accordance with claim 17 wherein there are N microwave
generators.
19. The apparatus in accordance with claim 18 including a mode partitioning
means which provides N outputs for each of said K audio frequency filters.
20. The apparatus in accordance with claim 19 wherein said N amplifiers
each have K inputs from said mode partitioning means.
21. The apparatus in accordance with claim 20 wherein said N amplifiers
have K inputs less the mode partitioning means outputs which are so small
that they may be omitted.
22. The apparatus in accordance with claim 20 wherein said mode
partitioning output device outputs each include a diode connected to each
microwave amplifier gain control to provide isolation between all outputs.
23. The apparatus in accordance with claim 20 wherein said K audio
frequency filters are chosen to correspond to the critical bandwidths of
the human ear.
24. The apparatus in accordance with claim 20 wherein said N microwave
generators are each adjustable in frequency output.
25. The apparatus in accordance with claim 18 wherein the frequency of each
N microwave generators is determined by anatomical estimation.
26. The apparatus in accordance with claim 18 wherein the frequency of the
lowest frequency microwave generator is chosen by determination of the
effect of external microwave generation on the EEG of the subject.
27. The apparatus in accordance with claim 18 wherein the frequency of each
of said N microwave generators corresponds to the subject's microwave
modal frequencies.
28. The apparatus in accordance with claim 27 wherein the subject's modal
frequencies are determined by measurement of the subject's cephalic index
and the lateral dimensions of the skull.
29. The apparatus in accordance with claim 28 wherein the subject's lowest
modal frequency is determined by varying the frequency of the lowest
frequency microwave generator about the estimated value until a maximum
acoustic perception is obtained by the subject.
Description
BACKGROUND OF THE INVENTION
1. Field of the Invention
This invention relates to devices for aiding of hearing in mammals. The
invention is based upon the perception of sounds which is experienced in
the brain when the brain is subjected to certain microwave radiation
signals.
2. Description of the Prior Art
In prior art hearing devices for human beings, it is well known to amplify
sounds to be heard and to apply the amplified sound signal to the ear of
the person wearing the hearing aid. Hearing devices of this type are
however limited to hearing disfunctions where there is no damage to the
auditory nerve or to the auditory cortex. In the prior art, if there is
damage to the auditory cortex or the auditory nerve, it cannot be
corrected by the use of a hearing aid.
During World War II, individuals in the radiation path of certain radar
installations observed clicks and buzzing sounds in response to the
microwave radiation. It was through this early observation that it became
known to the art that microwaves could cause a direct perception of sound
within a human brain. These buzzing or clicking sounds however were not
meaningful, and were not perception of sounds which could otherwise be
heard by the receiver. This type of microwave radiation was not
representative of any intelligible sound to be perceived. In such radar
installations, there was never a sound which was generated which resulted
in subsequent generation of microwave signals representative of that
sound.
Since the early perception of buzzing and clicking, further research has
been conducted into the microwave reaction of the brain. In an article
entitled "Possible Microwave Mechanisms of the Mammalian Nervous System"
by Philip L. Stocklin and Brain F. Stocklin, published in the TIT Journal
of Life Sciences, Tower International Technomedical Institute, Inc. P.O.
Box 4594, Philadelphia, Pa. (1979) there is disclosed a hypothesis that
the mammalian brain generates and uses electro magnetic waves in the lower
microwave frequency region as an integral part of the functioning of the
central and peripheral nervous systems. This analysis is based primarily
upon the potential energy of a protein integral in the neural membrane.
In an article by W. Bise entitled "Low Power Radio-Frequency and Microwave
Effects On Human Electroencephalogram and Behavior", Physiol. Chemistry
Phys. 10, 387 (1978), it is reported that there are significant effects
upon the alert human EEG during radiation by low intensity CW microwave
electromagnetic energy. Bise observed significant repeatable EEG effects
for a subject during radiation at specific microwave frequencies.
SUMMARY OF THE INVENTION
Results of theoretical analysis of the physics of brain tissue and the
brain/skull cavity, combined with experimentally-determined
electromagnetic properties of mammalian brain tissue, indicate the
physical necessity for the existence of electromagnetic standing waves,
called modes in the living mammalian brain. The mode characteristics may
be determined by two geometric properties of the brain; these are the
cephalic index of the brain (its shape in prolate spheroidal coordinates)
and the semifocal distance of the brain (a measure of its size). It was
concluded that estimation of brain cephalic index and semifocal distance
using external skull measurements on subjects permits estimation of the
subject's characteristic mode frequencies, which in turn will permit a
mode by mode treatment of the data to simulate hearing.
This invention provides for sound perception by individuals who have
impaired hearing resulting from ear damage, auditory nerve damage, and
damage to the auditory cortex. This invention provides for simulation of
microwave radiation which is normally produced by the auditory cortex. The
simulated brain waves are introduced into the region of the auditory
cortex and provide for perceived sounds on the part of the subject.
BRIEF DESCRIPTION OF THE DRAWINGS
FIG. 1 shows the acoustic filter bank and mode control matrix portions of
the hearing device of this invention.
FIG. 2 shows the microwave generation and antenna portion of the hearing
device of this invention.
FIG. 3 shows a typical voltage divider network which may be used to provide
mode partition.
FIG. 4 shows another voltage divider device which may be used to provide
mode partition.
FIG. 5 shows a voltage divider to be used as a mode partition wherein each
of the resistors is variable in order to provide adjustment of the voltage
outputs.
FIG. 6 shows a modified hearing device which includes adjustable mode
partitioning, and which is used to provide initial calibration of the
hearing device.
FIG. 7 shows a group of variable oscillators and variable gain controls
which are used to determine hearing characteristics of a particular
subject.
FIG. 8 shows a top view of a human skull showing the lateral dimension.
FIG. 9 shows the relationship of the prolate spherical coordinate system to
the cartesian system.
FIG. 10 shows a side view of a skull showing the medial plane of the head,
section A--A.
FIG. 11 shows a plot of the transverse electric field amplitude versus
primary mode number M.
FIG. 12 shows a left side view of the brain and auditory cortex.
FIG. 13 shows the total modal field versus angle for source location.
DETAILED DESCRIPTION OF THE PREFERRED EMBODIMENT
This invention is based upon observations of the physical mechanism the
mammalian brain uses to perceive acoustic vibrations. This observation is
based in part upon neuro anatomical and other experimental evidence which
relates to microwave brain stimulation and the perception of sounds.
It is has been observed that monochromatic acoustic stimuli (acoustic
tones, or single tones) of different frequencies uniquely stimulate
different regions of the cochlea. It has also been observed that there is
a corresponding one to one relationship between the frequency of a
monochromatic acoustic stimulus and the region of the auditory cortex
neurally stimulated by the cochlear nerve under the physiologically normal
conditions (tonotopicity).
It is has been observed that for an acoustic tone of a frequency which is
at the lower end of the entire acoustical range perceivable by a person,
that a thin lateral region ("Line") parallel to the medial axis of the
brain and toward the inferior portion of the primary auditory cortex is
stimulated. For an acoustic tone whose frequency is toward the high end of
the entire perceivable acoustic range, a thin lateral region parallel to
the medial axis and toward the superior portion of the primary auditory
cortex is stimulated.
Neural stimulation results in the generation of a broad band of microwave
photons by the change in rotational energy state of protons integral to
the neuron membrane of the auditory cortex. The physical size and shape of
the brain/skull cavity, together with the (semi-conductor) properties
(conductivity and dielectric constant) of the brain tissue provide an
electromagnetic resonant cavity. Specific single frequencies are
constructively reinforced so that a number of standing electromagnetic
waves, each at its own single electromagnetic frequency in the microwave
frequency region, are generated in the brain. Each such standing
electromagnetic wave is called a characteristic mode of the brain/skull
cavity.
Analysis in terms of prolate spheroidal wave functions indicates that
transverse electric field components of these modes have maxima in the
region of the auditory cortex. This analysis further shows that transverse
electric field possess a variation of amplitude with angle in the angular
plane (along the vertical dimension of the auditory cortex) and that is
dependent only upon the primary mode number.
The auditory cortex in the normally functioning mammalian brain is a source
of microwave modes. The auditory cortex generates these modes in
accordance with the neural stimulation of the auditory cortex by the
cochlear nerve. Mode weighting for any one acoustic tone stimulus is given
by the amplitude of each mode along the line region of the auditory cortex
which is neurally stimulated by that acoustic tone stimulus. A listing of
mode weighting versus frequency of acoustic stimulus is called the mode
matrix.
In this invention, the functions of the ear, the cochlear nerve, and the
auditory cortex are simulated. Microwaves simulating the mode matrix are
inserted directly into the region of the auditory cortex. By this
insertion of simulated microwave modes, the normal operation of the entire
natural hearing mechanism is simulated.
Referring now to FIG. 1 and FIG. 2 there is shown an apparatus which
provides for induced perception of sound into a mammalian brain. This
hearing device includes a microphone 10 which receives sounds, an acoustic
filter bank 12 which separates the signals from the microphone into
component frequencies, and a mode control matrix 14 which generates the
mode signals which are used to control the intensity of microwave
radiations which are injected into the skull cavity in the region of the
auditory. cortex.
The acoustic filter bank 12 consists of a bank of acoustic filters F1
through Fk which span the audible acoustic spectrum. These filters may be
built from standard resistance, inductance, and capacitance components in
accordance with well established practice. In the preferred embodiment
there are 24 filters which correspond to the observed critical bandwidths
of the human ear. In this preferred embodiment a typical list of filter
parameters is given by Table 1 below:
TABLE I
______________________________________
Filter No. Center Frequency (Hz)
Bandwidth (Hz)
______________________________________
1 50 less than 100
2 150 100
3 250 100
4 350 100
5 450 110
6 570 120
7 700 140
8 840 150
9 1,000 160
10 1,170 190
11 1,370 210
12 1,600 240
13 1,850 280
14 2,150 320
15 2,500 380
16 2,900 450
17 3,400 550
18 4,000 700
19 4,800 900
20 5,800 1,100
21 7,000 1,300
22 8,500 1,800
23 10,500 2,500
24 13,500 3,500
______________________________________
The rectifier outputs one through K are feed to K mode partition devices.
The mode partitioning devices each have N outputs wherein N is the number
of microwave oscillators used to generate the microwave radiation. The
outputs 1 through N of each mode partition device is applied respectively
to the inputs of each gain controlled amplifier of the microwave radiation
generator. The function of the mode control matrix 14 is the control of
the microwave amplifiers in the microwave amplifier bank 18. In the
preferred embodiment thus will be 24 outputs and 24 microwave frequency
oscillators.
Connected to each microwave amplifier gain control line is a mode
simulation device 16 which receives weighted mode signals from the mode
partition devices 14. Each mode simulation device consists of one through
k lines and diodes 17 which are each connected to summing junction 19. The
diodes 17 provide for isolation from one mode partition device to the
next. The diodes 17 prevent signals from one mode partition device from
returning to the other mode partition devices which are also connected to
the same summing junction of the mode summation device 16. The diodes also
serve a second function which is the rectification of the signals received
from the acoustic filter bank by way of the mode partition devices. In
this way each mode partition device output is rectified to produce a
varying DC voltage with major frequency components of the order of 15
milliseconds or less. The voltage at the summation junction 19 is thus a
slowly varying DC voltage.
The example mode partition devices are shown in greater detail in FIGS. 3,
4, and 5. The mode partition devices are merely resistance networks which
produce 1 through N output voltages which are predetermined divisions of
the input signal from the acoustic filter associated with the mode
partition device. FIG. 3 shows a mode partitioning device wherein several
outputs are associated with each series resistor 30. In the embodiment
depicted in FIG. 4 there is an output associated with each series resistor
only, and thus there are N series resistors, or the same number of series
resistors as there are outputs. The values of the resistors in the mode
partition resistor network are determined in accordance with the
magnitudes of the frequency component from the acoustic filter bank 12
which is required at the summation point 19 or the gain control line for
amplifiers 20.
The microwave amplifier bank 18 consists of a plurality of microwave
oscillators 1 through N each of which is connected to an amplifier 20.
Since the amplifiers 20 are gain controlled by the signals at summation
junction 19, the magnitude of the microwave output is controlled by the
mode control matrix outputs F1 through F.sub.n. In the preferred
embodiment there are 24 amplifiers.
The leads from the microwave oscillators 1 through N to the amplifiers 20
are shielded to prevent cross talk from one oscillator to the next, and to
prevent stray signals from reaching the user of the hearing device. The
output impedance of amplifiers 20 should be 1000 ohms and this is
indicated by resistor 21. The outputs of amplifiers 20 are all connected
to a summing junction 22. The summing junction 22 is connected to a
summing impedance 23 which is approximately 50 ohms. The relatively high
amplifier output impedance 21 as compared to the relatively low summing
impedance 23 provides minimization of cross talk between the amplifiers.
Since the amplitude of the microwave signal needed at the antenna 24 is
relatively small, there is no need to match the antenna and summing
junction impedances to the amplifier 20 output impedances. Efficiency of
the amplifiers 20 is not critical.
Level control of the signal at antenna 24 is controlled by pick off 25
which is connected to the summing impedance 23. In this manner, the signal
at antenna 24 can be varied from 0 (ground) to a value which is acceptable
to the individual.
The antenna 24 is placed next to the subject's head and in the region of
the subject's auditory cortex 26. By placement of the antenna 24 in the
region of the auditory cortex 26, the microwave field which is generated
simulates the microwave field which would be generated if the acoustic
sounds were perceived with normal hearing and the auditory cortex was
functioning normally.
In FIG. 2A there is shown a second embodiment of the microwave radiation
and generator portion of the hearing device. In this embodiment a broad
band microwave source 50 generates microwave signals which are feed to
filters 52 through 58 which select from the broad band radiation
particular frequencies to be transmitted to the person. As in FIG. 2, the
amplifiers 20 receive signals on lines 19 from the mode control matrix.
The signals on lines 19 provide the gain control for amplifiers 20.
In FIG. 6 there is shown a modified microwave hearing generator 60 which
includes a mode partition resistor divider network as depicted in FIG. 5.
Each of the mode partition voltage divider networks in this embodiment are
individually adjustable for all of the resistances in the resistance
network. FIG. 5 depicts a voltage division system wherein adjustment of
the voltage partition resistors is provided for.
In FIG. 6, the sound source 62 generates audible sounds which are received
by the microphone of the microwave hearing generator 60. In accordance
with the operation described with respect to FIGS. 1 and 2, microwave
signals are generated at the antenna 10 in accordance with the
redistribution provided by the mode control matrix as set forth in FIG. 5.
The sound source 62 also produces a signal on line 64 which is received by
a head phone 66. The apparatus depicted in FIG. 6 is used to calibrate or
fit a microwave hearing generator to a particular individual. Once the
hearing generator is adjusted to the particular individual by adjustment
of the variable resistors in the adjustable mode partition portion of the
hearing generator, a second generator may be built using fixed value
resistors in accordance with the adjusted values achieved in fitting the
device to the particular subject. The sound produced by headphone 66
should be the same as a sound from the sound source 62 which is received
by the microphone 10 in the microwave hearing generator 60. In this way,
the subject can make comparisons between the perceived sound from the
hearing generator 60, and the sound which is heard from headphone 66.
Sound source 62 also produces a signal on 68 which is feed to cue light
69. Cue light 69 comes on whenever a sound is emitted from sound source 62
to the microwave generator 60. In this manner, if the subject hears
nothing, he will still be informed that a sound has been omitted and hence
that he is indeed perceiving no sound from the microwave hearing generator
60.
In FIG. 7 there is shown a modified microwave hearing generator which may
be used to determine a subject's microwave mode frequencies. In this
device, the acoustic filter bank and the mode control matrix have been
removed and replaced by voltage level signal generated by potentiometers
70. Also included are a plurality of variable frequency oscillators 72
which feed microwave amplifiers 74 which are gain controlled from the
signal generated by potentiometers 70 and pick off arm 76.
This modified microwave hearing generator is used to provide signals using
one oscillator at a time. When an oscillator is turned on, the frequency
is varied about the estimated value until a maximum acoustic perception by
the subject is perceived. This perception however may consist of a buzzing
or hissing sound rather than a tone because only one microwave frequency
is being received. The first test of perception is to determine the
subject's lowest modal frequency for audition (M=1). Once this modal
frequency is obtained, the process is repeated for several higher modal
frequencies and continued until no maximum acoustic perception occurs.
Another method of determination of a subject's modal frequencies is through
anatomical estimation. This procedure is by measurement of the subject's
cephalic index and the lateral dimensions of the skull. In this method,
the shape is determined in prolate spheroidal coordinance.
Purely anatomical estimation of subject's modal frequencies is performed by
first measuring the maximum lateral dimension (breadth) L FIG. 8, of the
subject's head together with the maximum dimension D (anterior to
posterior) in the medial plane of the subject's head. D is the distance
along Z axis as shown in FIG. 10. The ratio L/D, called in anthropology
the cephalic index, is monotonically related to the boundary value
.xi..sub.o defining the ellipsoidal surface approximating the interface
between the brain and the skull in the prolate spheroidal coordinate
system. .xi..sub.o defines the shape of this interface; .xi..sub.o and D
together give an estimate of a, the semi-focal distance of the defining
ellipsoid. Using .xi..sub.o and a, together with known values of the
conductivity and dielectric constants of brain tissue, those wavelengths
are found for which the radial component of the electric field satisfies
the boundary condition that it is zero at .xi..sub.o. These wavelengths
are the wavelengths associated with the standing waves or modes; the
corresponding frequencies are found by dividing the phase velocity of
microwaves in brain tissue by each of the wavelengths.
A subject's microwave modal frequencies may also be determined by observing
the effect of external microwave radiation upon the EEG. The frequency of
the M equal 1 mode may then be used as a base point to estimate all other
modal frequencies.
A typical example of such an estimation is where the subject is laterally
irradiated with a monochromatic microwave field simultaneous with EEG
measurement and the microwave frequency altered until a significant change
occurs in the EEG, the lowest such frequency causing a significant EEG
change is found. This is identified as the frequency of the M=1 mode, the
lowest mode of importance in auditory perception. The purely anatomical
estimation procedure (FIGS. 8, 9, 10) is then performed and the ratio of
each modal frequency to the M=1 modal frequency obtained. These ratios
together with the experimentally-determined M=1 frequency are then used to
estimate the frequencies of the mode numbers higher than 1. The prolate
spheroidal coordinate system is shown in FIG. 9. Along the lateral plane
containing the x and y coordinates of FIG. 9, the prolate spheroidal
coordinate variable .phi. (angle) lies FIGS. 9 and 10. Plots of the
transverse electric field amplitude versus primary mode number m are shown
in FIG. 11. The equation is
E.sub.transverse (m, .phi.)=E.sub.o sin(m .phi.)
The "elevation view" FIG. 12, of the brain from the left side, shows the
primary auditory cortex 10. The iso-tone lines and the high frequency
region are toward the top of 100 and the low frequency region toward the
bottom of 100.
The formula I, set forth below is the formula for combining modes from an
iso-tone line at .phi.=.phi.j being excited to obtain the total modal
field at some other angular location .phi.. For this formula, if we let
J=1 (just one iso-tone single frequency acoustic stimulus line), then it
can be shown that ALL modes (in general) must be used for any ONE tone.
##EQU1##
.phi.=ANGLE (0.degree. LATERAL) .phi..sub.j =LOCATION OF j-TH SOURCE
(TOTAL NUMBER J)
.DELTA..phi..sub.m =ATTENUATION LENGTH (IN ANGLE) OF m-TH MODE
m=PRIMARY MODE NUMBER (HIGHEST MODE M)
FIG. 13 shows the resulting total modal field versus angle .phi. for source
location .phi. at 5.25.degree., 12.5.degree., etc. With reference to the
set of curves at the left top of this figure. A spacing of approximately
7.25.degree. in .phi. corresponds to a tonal difference of about 1 octave.
This conclusion is based on the side-lobes of pattern coming from
.phi.=5.25.degree., etc. The total filed (value on y-axis) falls
considerably below the top curves for source locations well below
5.25.degree. (toward the high acoustic stimulus end) and also as the
source of frequency goes well above 30.degree. (low frequency end). .phi.
is plotted positive downward from 0.degree. at lateral location as
indicates in FIG. 11.
Resistor weightings are obtained from the .vertline.sin
(m[.phi.-.phi.j]).vertline., Formula I. The scale between acoustic
frequency and .phi. must be set or estimated from experiment.
Approximately 5.25.+-.1.degree. corresponds to a tonal stimulus at about 2
kHz (the most sensitive region of the ear) since this source location
gives the highest electric field amplitude.
The apparatus of FIG. 7 may also be used to determine values for a hearing
device which are required for a particular subject. Once the modal
frequencies have been estimated, the device of FIG. 7 which includes
variable microwave oscillators may be used to determine values for the
oscillators which match the subject, and to determine resistance values
associated with the mode partition devices of the mode control matrix.
In FIG. 7 manual control of the amplifier gain is achieved by
potentiometers 76. In this manner the amplifier gains are varied about the
estimated settings for an acoustic tone stimulus in the region of two
thousand Hertz (2 kHz) until maximum acoustic perception and a purest tone
are achieved together. The term purest tone may also be described as the
most pleasing acoustic perception by the subject. This process may be
repeated at selected frequencies above and below 2 kHz. The selected
frequencies correspond to regions of other acoustic filter center
frequencies of the subject. When modal frequency (oscillator frequency)
and gain set values (setting a potentiometer 76) are noted, it is then
possible to calculate fixed oscillator frequencies and control resistor
values for the adjusted hearing device for this particular subject.
In the event the subject has no prior acoustic experience, that is deaf
from birth, estimated resistor values must be used. Also, a complex
acoustic stimulation test including language articulation and pairs of
harmonically related tones may be developed to maximize the match of the
hearing device parameters for those of this particular subject.
Typical components for use in this invention include commercially available
high fidelity microphones which have a range of 50 Hz to 15 kHz with plus
or minus 3 dB variation.
The audio filters to be used with the acoustic filter bank 12 are
constructed in a conventional manner, and have Q values of about 6. The
filters may also be designed with 3 dB down points (1/2 the bandwidth away
from the center frequency) occurring at adjacent center frequency
locations.
The diodes 17 in the mode control matrix which provide isolation between
the mode partition circuits are commercially available diodes in the audio
range.
The microwave oscillators 1 through N and the microwave amplifiers 20 are
constructed with available microwave transistors which can be configured
either as oscillators or amplifiers. Examples of the transistors are
GaAsFET field effect transistors by Hewlitt Packard known as the HFET
series or silicone bipolar transistors by Hewlitt Packard known as the
HXTR series.
All the cable between the oscillators, the microwave amplifiers, and the
antenna should be constructed with either single or double shielded
coaxial cable.
The antenna 24 for directing microwave signals to the audio cortex 26
should be approximately the size of the auditory cortex. A typical size
would be one and one half CM high and one half to one CM wide. The antenna
as shown is located over the left auditory cortex, but the right may also
be used. Since the characteristic impedance of the brain tissue at these
microwave frequencies is close to 50 ohms, efficient transmission by
commercially available standard 50 ohm coax is possible.
The invention has been described in reference to the preferred embodiments.
It is, however, to be understood that other advantages, features, and
embodiments may be within the scope of this invention as defined in the
appended claims.
* * * * *
TOP
Return To Microwave Detection | Info | Home
 760-668-2245
Do Not Text
Email: [email protected]
Over 30 years of specialized service
over 2,500 locations swept.
PLEASE DO NOT CONTACT US
FROM A SUSPECT TELEPHONE OR FACILITY
WHEN INQUIRING ABOUT BUG SWEEPING OR OUR
SERVICES AND SURVEYS.
|
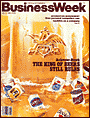
Read about us in
Business Week |